What if you could explore every gene, protein, and molecule in a living organism to uncover the secrets of life? That’s exactly what omics allows scientists to do. By studying biological systems on a massive scale, omics has transformed the way we understand genetics, diseases, and even ecosystems.
From genomics and proteomics to cutting-edge multi-omics approaches, this article provides a comprehensive overview of omics—explaining its foundations, recent advancements, and its potential to revolutionize science and medicine. Whether you’re curious about what omics is or how it shapes modern research, you’re in the right place.
What Is Omics? A Simple Explanation for Everyone
Omics is the large-scale study of biological molecules that are essential for life. Unlike traditional approaches that focus on individual components, omics provides a holistic view of an organism by analyzing its genetic, molecular, and metabolic systems. This broad perspective allows scientists to uncover how these components interact and contribute to health, disease, and environmental responses.
The Building Blocks of Omics
The term “omics” refers to the comprehensive study of biological “omes,” which represent complete sets of molecules within a system. For instance, genomics examines the entire genome, the DNA blueprint that dictates an organism’s characteristics. Moving beyond DNA, transcriptomics focuses on RNA, which acts as the messenger that translates genetic information into proteins. Proteomics studies proteins, the functional molecules that drive biological processes, while metabolomics explores metabolites, the small molecules that provide real-time snapshots of cellular activity. Together, these fields create a layered understanding of biology.
Why Omics Matters
The importance of omics lies in its ability to connect the dots across biological systems. In medicine, omics has been instrumental in identifying genetic mutations linked to diseases and developing personalized treatments tailored to an individual’s unique biology. In agriculture, it enables the design of crops that can withstand extreme conditions, such as drought or pests. Similarly, in environmental science, omics approaches like microbiomics have revealed how microbial communities regulate ecosystems and influence climate change.
Omics in Everyday Terms
Imagine omics as creating a detailed map of everything happening inside a living system. Instead of analyzing isolated pieces, it provides a complete picture of how genes, proteins, and metabolites work together to sustain life. This systems-wide approach is what makes omics such a powerful tool for tackling complex scientific and societal challenges.
Foundations of Omics: The Core Disciplines
Omics serves as the backbone of modern biological research, with several core disciplines providing unique insights into the inner workings of living organisms. Each field focuses on a specific layer of biological information, contributing to a comprehensive understanding of life’s complexity.
Genomics: Decoding the Blueprint of Life
Genomics is the study of an organism’s complete DNA sequence, known as its genome. It focuses on identifying genes, understanding their functions, and unraveling how they interact with each other and the environment. Advances in high-throughput sequencing technologies, such as next-generation sequencing (NGS), have revolutionized genomics, enabling rapid and cost-effective analysis of entire genomes. These advancements have led to breakthroughs in fields like personalized medicine, where a patient’s genetic profile is used to develop tailored treatments.
Epigenomics: Beyond the DNA Sequence
While genomics deciphers the static blueprint of DNA, epigenomics investigates the chemical modifications that regulate gene expression without altering the underlying DNA sequence. These modifications, such as DNA methylation and histone modifications, act as “switches” that turn genes on or off in response to environmental factors. Epigenomics provides crucial insights into processes like development, aging, and disease. For example, abnormal epigenetic patterns have been linked to cancer and other chronic conditions. Advances in bisulfite sequencing and chromatin immunoprecipitation (ChIP-seq) have made it possible to map these modifications with high precision.
Transcriptomics: Understanding Gene Expression
While genomics reveals the DNA blueprint, transcriptomics examines how genes are expressed through RNA. By studying RNA molecules, transcriptomics provides insight into which genes are active under specific conditions, such as during disease progression or environmental stress. Technologies like RNA sequencing (RNA-seq) have made it possible to capture dynamic snapshots of gene activity, helping researchers identify critical pathways involved in cellular responses.
Read more: Bulk RNA-Seq Data Analysis
Proteomics: Uncovering Cellular Machinery
Proteomics takes the next step by studying proteins, the molecules responsible for most biological functions. Unlike DNA or RNA, proteins are highly dynamic, with their structure and activity changing in response to cellular needs. Mass spectrometry has become a cornerstone of proteomics, allowing for precise identification and quantification of proteins. This discipline is crucial for understanding diseases at the molecular level, as it sheds light on dysfunctional proteins that may drive conditions like cancer or neurodegenerative disorders.
Metabolomics: Capturing Cellular Metabolism
Metabolomics focuses on small molecules called metabolites, which are produced during metabolic processes. These metabolites provide a real-time snapshot of an organism’s physiological state. For example, shifts in metabolic profiles can indicate early signs of disease or reveal how an organism adapts to environmental changes. Advanced tools, such as nuclear magnetic resonance (NMR) spectroscopy and high-resolution mass spectrometry, are used to analyze metabolite concentrations with incredible precision, making metabolomics a key player in diagnosing diseases and optimizing therapies.
How These Core Disciplines Interconnect
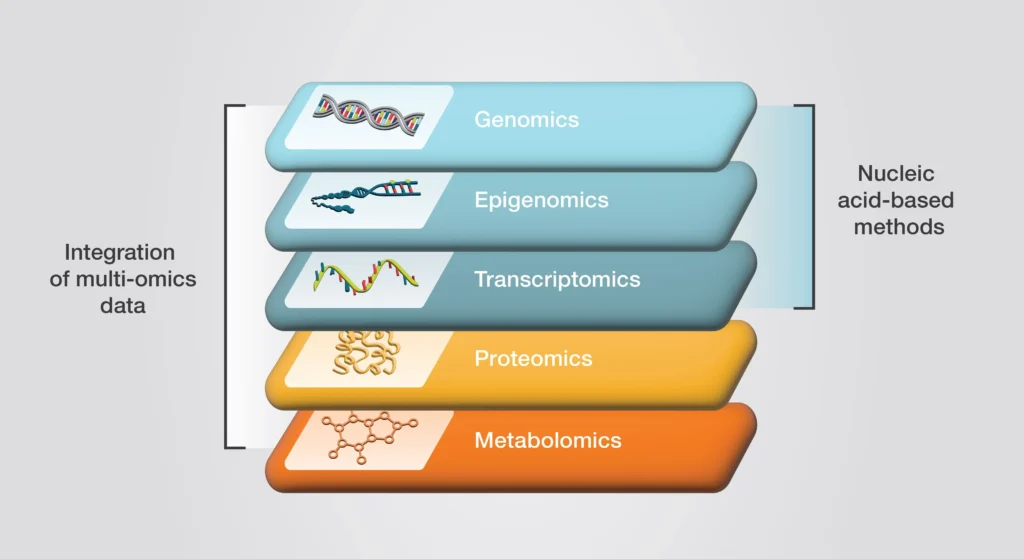
Each omics discipline provides a unique perspective on biology, but their power lies in their integration. Genomics lays the foundation by revealing the DNA blueprint, while epigenomics adds regulatory layers that control gene activity. Transcriptomics tracks dynamic gene expression, proteomics examines the functional molecules driving cellular processes, and metabolomics reflects the organism’s biochemical output. Together, these fields enable researchers to understand biological systems as interconnected networks, revolutionizing our ability to diagnose diseases, develop treatments, and address global challenges in health and the environment.
Beyond the Basics: Emerging Omics Disciplines
While the core omics disciplines—genomics, transcriptomics, proteomics, metabolomics, and epigenomics—provide the foundation for understanding biological systems, emerging omics fields are pushing the boundaries of what we can study. These new disciplines focus on specialized aspects of biology, offering deeper insights into molecular interactions and systems biology.
1. Interactomics: Mapping Molecular Relationships
Interactomics is the study of interactions within biological systems, including protein-protein, protein-DNA, and RNA-protein interactions. By mapping these networks, interactomics provides a detailed picture of how molecules work together to regulate cellular functions.
- Applications:
- Understanding protein-protein interactions to identify drug targets in diseases like cancer.
- Exploring RNA-protein networks to uncover mechanisms of gene regulation in neurodegenerative disorders.
Advances in technologies like yeast two-hybrid systems, cross-linking mass spectrometry, and RNA immunoprecipitation have made it possible to study these complex networks at unprecedented resolution.
2. Microbiomics: Exploring Microbial Communities
Microbiomics focuses on studying the genomes and interactions of microbial communities, such as those in the human gut or in environmental ecosystems. The microbiome plays a critical role in health, influencing everything from digestion to immune function.
- Applications:
- Understanding the gut microbiome’s impact on diseases like inflammatory bowel disease and obesity.
- Investigating the role of soil microbiomes in crop health and sustainable agriculture.
Next-generation sequencing and metagenomic analysis are key tools in microbiomics, allowing researchers to explore microbial diversity and function in complex environments.
3. Glycomics: Decoding Sugar Molecules
Glycomics examines glycans, the complex sugar molecules attached to proteins and lipids. Glycans are critical for cellular communication, immune responses, and pathogen recognition, yet they remain one of the least understood areas of biology.
- Applications:
- Investigating how glycan structures influence viral infections, such as in HIV or influenza.
- Developing glycan-based biomarkers for cancer diagnostics.
Advanced techniques like glycan mass spectrometry and glycoengineering are helping uncover the roles of these intricate molecules.
4. Lipidomics: Understanding Lipid Profiles
Lipidomics focuses on lipids, a diverse group of molecules essential for energy storage, membrane structure, and signaling. This field sheds light on how lipids regulate cellular processes and contribute to diseases like cardiovascular disorders and diabetes.
- Applications:
- Profiling lipid changes in metabolic diseases to identify therapeutic targets.
- Investigating the role of lipid metabolism in neurodegenerative diseases like Alzheimer’s.
Mass spectrometry and chromatography are pivotal tools for characterizing lipid composition and dynamics.
5. Redoxomics: Investigating Cellular Redox States
Redoxomics studies the balance of oxidants and antioxidants in cells, focusing on redox reactions that influence cellular signaling and decision-making. Disruptions in redox balance are linked to aging, cancer, and neurodegenerative diseases.
- Applications:
- Exploring how oxidative stress contributes to diseases like Parkinson’s and ALS.
- Developing antioxidant therapies to restore redox balance in pathological conditions.
Techniques like redox proteomics and fluorescence-based sensors enable detailed analysis of redox states within cells.
The Importance of Emerging Omics Fields
These emerging omics disciplines are not isolated—they complement the core omics fields by adding new dimensions to our understanding of biology. For example, interactomics can connect findings from proteomics and transcriptomics, while lipidomics and glycomics provide critical context for metabolic and signaling pathways. Together, they create a more complete picture of life’s complexity, paving the way for new discoveries in health, disease, and beyond.
Advancements in Omics Technologies
The evolution of omics technologies has transformed our ability to study biological systems at an unprecedented scale and resolution. From breakthroughs in sequencing to innovations in imaging and computational tools, these advancements are enabling researchers to tackle complex questions with greater precision and efficiency. Here’s an overview of the most impactful technological strides in omics.
1. Sequencing Technologies: From Sanger to Single Molecules
The development of DNA sequencing technologies is one of the most significant advancements in omics. What began with Sanger sequencing in the 1970s has progressed to next-generation sequencing (NGS) and, more recently, third-generation sequencing (TGS).
- Sanger Sequencing: The foundational method for determining DNA sequences, suitable for small-scale projects.
- Next-Generation Sequencing (NGS): Revolutionized genomics by enabling high-throughput analysis, drastically reducing time and cost. NGS is now used widely for applications like genome-wide association studies and RNA sequencing.
- Third-Generation Sequencing (TGS): Techniques like PacBio’s SMRT (Single Molecule, Real-Time) sequencing and Oxford Nanopore sequencing offer long-read capabilities. These methods are particularly valuable for resolving structural variants, mapping epigenetic modifications, and studying complex genomes.
Impact: These advancements have expanded the scope of genomics and epigenomics, allowing researchers to explore genetic and epigenetic changes with remarkable detail.
2. High-Resolution Mass Spectrometry in Proteomics and Metabolomics
Mass spectrometry (MS) has undergone substantial advancements, becoming a cornerstone for proteomics and metabolomics. Modern MS technologies offer exceptional sensitivity and resolution, enabling the detailed analysis of complex biological samples.
- Orbitrap and FT-ICR Mass Spectrometry: Provide ultra-high resolution and accuracy for identifying proteins and metabolites.
- Tandem Mass Spectrometry (MS/MS): Allows researchers to characterize protein structures and modifications, such as phosphorylation or glycosylation.
- Advances in Sample Preparation: Techniques like laser capture microdissection and isobaric labeling improve the accuracy and efficiency of MS workflows.
Impact: These innovations are crucial for studying protein interactions, metabolic pathways, and disease biomarkers, advancing our understanding of cellular functions and disease mechanisms.
3. Single-Cell and Spatial Omics Technologies
The ability to study individual cells and their spatial organization within tissues has opened new frontiers in omics. Single-cell and spatial omics technologies provide unprecedented resolution, revealing cellular heterogeneity and tissue architecture.
- Single-Cell RNA Sequencing (scRNA-seq): Captures gene expression profiles of individual cells, uncovering rare cell types and states.
- Spatial Transcriptomics: Combines gene expression data with spatial context, allowing researchers to map where genes are active within tissues.
- Multi-Modal Single-Cell Analysis: Integrates data from multiple omics layers (e.g., RNA, proteins, and metabolites) at the single-cell level.
Impact: These tools are transforming fields like immunology and cancer research by revealing how cellular environments influence behavior and function.
4. Computational and Data Integration Tools
As omics technologies generate vast datasets, computational tools are essential for managing, analyzing, and integrating this information. Recent advancements in bioinformatics and machine learning have enhanced data processing capabilities.
- Cloud Computing: Platforms like AWS and Google Cloud enable the storage and analysis of massive omics datasets.
- AI and Machine Learning: Predictive models identify patterns across multi-omics datasets, improving insights into complex systems.
- Data Integration Frameworks: Tools like Cytoscape and iCluster facilitate the combination of genomics, transcriptomics, proteomics, and other omics data.
Impact: These advancements help researchers uncover new insights from multi-dimensional data, leading to breakthroughs in systems biology and personalized medicine.
How These Advancements Are Driving Omics Forward
The rapid pace of technological innovation in omics is reshaping how scientists study life. Sequencing technologies have made genomics and epigenomics more accessible, while high-resolution mass spectrometry enhances proteomics and metabolomics. Meanwhile, single-cell and spatial omics tools provide unparalleled insights into cellular diversity and organization. Coupled with advances in computational methods, these technologies are enabling a deeper, more integrated understanding of biological systems, ultimately driving progress in medicine, agriculture, and environmental science.
Applications of Omics in Science and Medicine
Omics technologies are reshaping science and medicine by providing unprecedented insights into biological systems. By analyzing genes, proteins, and metabolites at a large scale, omics enables scientists to uncover intricate mechanisms underlying health and disease, personalize treatments, and address environmental challenges. Below, we explore how omics is applied across key domains with detailed descriptions of their transformative impact.
1. Personalized Medicine: Tailoring Treatments to Individuals
Personalized medicine leverages omics data to design treatments that align with an individual’s unique biological profile. By combining insights from genomics, proteomics, and metabolomics, researchers can identify the molecular drivers of disease and predict how a patient will respond to specific therapies.
For example, in cancer treatment, genomic sequencing can identify mutations in genes like BRCA1 and BRCA2, which are associated with higher risks of breast and ovarian cancers. These insights guide clinicians in choosing preventive measures or targeted therapies, such as PARP inhibitors. Proteomics adds another layer by analyzing the proteins produced in tumors, highlighting potential biomarkers for tracking disease progression. Meanwhile, metabolomics helps monitor the metabolic shifts caused by cancer, enabling adjustments to treatment regimens in real time.
The integration of multi-omics data is particularly powerful. In cardiovascular disease, for instance, combining genomic data with metabolomic profiles has led to the discovery of biomarkers that predict heart attack risks more accurately than traditional methods. This approach improves treatment outcomes, reduces adverse effects, and lowers healthcare costs by focusing resources where they’re most needed.
2. Understanding Complex Diseases
Many diseases, such as cancer, Alzheimer’s, and diabetes, result from complex interactions between genetic, molecular, and environmental factors. Omics technologies provide a systems-level view, allowing researchers to dissect these complexities and identify critical pathways driving disease.
In cancer research, multi-omics studies are uncovering how mutations in DNA interact with changes in RNA expression, protein function, and metabolism to drive tumor growth and metastasis. For example, in triple-negative breast cancer, transcriptomic and proteomic analyses have identified key pathways that are now being targeted by novel drugs. Omics also enables the stratification of patients into subgroups based on their molecular profiles, allowing for more precise treatments.
In neurodegenerative diseases like Alzheimer’s, proteomics has identified abnormal protein aggregates, such as beta-amyloid and tau, that are hallmarks of the disease. Metabolomics complements this by highlighting metabolic changes in the brain, which could serve as early warning signs before clinical symptoms appear. This integrated approach has led to the development of potential biomarkers for early diagnosis and monitoring disease progression.
Similarly, in diabetes research, metabolomics has been instrumental in understanding the metabolic imbalances that drive insulin resistance. By linking these findings with genomic data, researchers are identifying new drug targets and tailoring dietary interventions to individual metabolic profiles.
3. Microbiomics and Environmental Applications
The study of microbial communities—whether in the human body, soil, or aquatic environments—is another major application of omics technologies. Microbiomics focuses on understanding the diversity, interactions, and functions of these microorganisms, which play crucial roles in health and ecosystems.
In human health, the gut microbiome has been linked to a wide range of conditions, including obesity, inflammatory bowel disease, and even mental health disorders like depression. Omics technologies, such as metagenomics, allow researchers to profile the composition of microbial communities and understand how they influence host biology. For example, analyzing the gut microbiota has revealed specific bacterial strains that promote better digestion or modulate immune responses.
In agriculture, soil microbiomics helps improve crop yields and resilience by studying how microbial communities contribute to nutrient cycling and disease resistance. By applying omics to soil health, farmers can reduce their reliance on chemical fertilizers and pesticides, making farming more sustainable.
Environmental applications are equally significant. Metagenomics and metabolomics are used to monitor ecosystems and detect pollutants. For instance, tracking microbial communities in water systems can reveal the presence of toxic substances or predict the effects of climate change on biodiversity. These insights are critical for designing interventions that protect ecosystems and promote environmental sustainability.
4. Drug Discovery and Development
Omics technologies are accelerating drug discovery by identifying new therapeutic targets and optimizing drug candidates. Genomics and proteomics are at the forefront of this process, providing detailed insights into the molecular mechanisms of diseases.
In early drug discovery, genomic studies identify genes associated with diseases, while proteomic analyses reveal the proteins they produce, which often serve as drug targets. For example, in autoimmune diseases, proteomic studies have pinpointed cytokines that drive inflammation, leading to the development of targeted biologics like monoclonal antibodies.
Metabolomics plays a crucial role in understanding how drugs interact with the body. By profiling metabolic changes, researchers can predict potential side effects and refine drug formulations to minimize toxicity. Additionally, multi-omics approaches enable the simultaneous evaluation of efficacy and safety, reducing the time and cost of clinical trials.
Drug repurposing is another area where omics excels. By analyzing existing drugs against omics datasets, researchers have discovered new applications for old compounds. For instance, metformin, originally developed for diabetes, has shown potential in cancer treatment due to its effects on metabolic pathways uncovered through metabolomic studies.
Omics: A Catalyst for Scientific and Medical Advancements
Omics technologies are driving transformative advancements across science and medicine by offering detailed insights into biological systems. In personalized medicine, they enable tailored treatments that improve outcomes and reduce costs. In the study of complex diseases, they unravel intricate molecular networks, paving the way for new diagnostics and therapies. Applications in microbiomics and environmental science highlight their potential to address global health and ecological challenges. Finally, in drug discovery, omics accelerates the development of safer and more effective therapies. By integrating these disciplines, researchers are unlocking the full potential of biological systems, setting the stage for a healthier and more sustainable future.
Challenges and Future Directions in Omics
Omics technologies have changed how we study biology, but they also come with challenges that researchers must address. At the same time, the future of omics holds exciting opportunities to improve how we analyze and apply biological data.
Challenges in Omics
- Handling Large and Complex Data
Omics studies produce massive amounts of data, often from multiple sources like DNA, RNA, proteins, and metabolites. Combining these datasets to get a complete picture of biological systems is complicated because each type of data requires different methods and tools. Additionally, ensuring the quality of the data and filtering out errors is an ongoing challenge. - Limited Computational Tools
Analyzing omics data demands powerful computers and specialized software. Many researchers, especially those in smaller labs or resource-limited areas, don’t have access to these tools. Developing better algorithms and making them more accessible will be crucial for advancing the field. - Inconsistent Methods and Results
Different labs use different protocols for collecting and analyzing omics data, which can lead to inconsistent results. Without standard practices, it’s hard to compare findings or confirm their accuracy, especially for studies that need to be replicated for clinical use. - Accessibility Issues
Advanced omics technologies, like sequencing machines and mass spectrometers, are expensive. This makes it difficult for researchers in less-funded regions to participate fully in omics research, creating gaps in global scientific efforts.
Future Directions in Omics
- Using AI for Smarter Data Analysis
Artificial intelligence and machine learning are becoming essential tools in omics. They can help researchers analyze complex datasets more efficiently and find patterns that might otherwise go unnoticed. For example, AI can combine data from multiple omics layers to predict how diseases develop or how patients might respond to treatments. - Improving Multi-Omics Integration
New tools are being developed to combine data from different omics fields into a single, unified analysis. This approach will make it easier to study how genes, proteins, and metabolites interact in complex systems like cancer or aging. Cloud-based platforms and open-source software will also make these tools available to more researchers. - Expanding into New Omics Areas
Beyond genomics and proteomics, newer fields like lipidomics (the study of fats) and glycomics (the study of sugar molecules) are gaining attention. Adding these layers to existing omics research will help answer questions about how different molecules work together in health and disease. - Making Omics Technologies More Accessible
Efforts are underway to create more affordable and portable omics tools. For instance, compact sequencing devices are making it possible to study DNA in remote locations or small labs. These advancements will help more researchers participate in omics studies, regardless of their resources. - Addressing Ethical Concerns
As omics becomes more widely used, privacy and ethical concerns are growing. It’s important to ensure that personal data, especially in healthcare, is handled responsibly and with proper consent. Clear guidelines and international cooperation will help build trust and fairness in how omics data is used.
Looking Ahead
While challenges like data complexity, cost, and standardization remain, the future of omics is full of possibilities. With better tools, smarter analysis methods, and wider access, researchers can continue to uncover how biological systems work and use that knowledge to improve healthcare, agriculture, and the environment. By addressing these challenges thoughtfully, omics will play an even bigger role in shaping the future of science and medicine.
FAQs About Omics
- What is omics, and why is it important?
Omics refers to the large-scale study of biological molecules, such as genes, proteins, and metabolites, to understand how they interact within a system. It’s important because it provides a holistic view of biology, helping researchers uncover the molecular mechanisms of health, disease, and environmental changes. - How is omics used in personalized medicine?
In personalized medicine, omics data—such as genomics, proteomics, and metabolomics—is used to tailor treatments to an individual’s biological profile. For example, genetic testing can identify mutations that influence drug responses, enabling doctors to prescribe therapies that are more effective and have fewer side effects. - What is the difference between genomics and multi-omics?
Genomics focuses specifically on the study of DNA, while multi-omics integrates data from multiple fields, such as transcriptomics (RNA), proteomics (proteins), and metabolomics (metabolites). Multi-omics provides a more comprehensive understanding of how these layers work together in complex biological systems. - What are the main challenges in omics research?
Key challenges include managing and analyzing large datasets, integrating data from different omics fields, ensuring reproducibility across studies, and addressing the high costs of advanced technologies. These issues make it difficult to fully utilize omics for widespread research and clinical applications. - How can omics technologies address global health issues?
Omics can improve global health by enabling early disease detection, identifying new drug targets, and developing vaccines. For instance, during the COVID-19 pandemic, genomics played a crucial role in tracking virus mutations and guiding vaccine development. However, making these technologies more accessible globally is essential to ensure equitable benefits.
Conclusion: Unlocking the Power of Omics
Omics has revolutionized our understanding of biology by providing a systems-wide view of the molecules that drive life. From genomics and proteomics to emerging disciplines like lipidomics and microbiomics, these technologies are reshaping science and medicine. They have enabled personalized treatments, uncovered the molecular mechanisms of complex diseases, and advanced our ability to monitor and protect the environment.
Despite the challenges of managing large datasets, ensuring reproducibility, and addressing accessibility gaps, ongoing innovations are paving the way for a more inclusive and impactful future. Advances in artificial intelligence, multi-omics integration, and affordable technologies are making it easier for researchers worldwide to harness the full potential of omics.
As we move forward, collaboration, ethical responsibility, and continued innovation will be key to unlocking even greater discoveries. Omics is not just transforming how we study life—it’s shaping how we improve health, sustain the environment, and solve the most pressing challenges of our time. Now is the moment to embrace the possibilities and drive the future of science and medicine with the power of omics.
References
- Vailati-Riboni, M., Palombo, V., Loor, J.J. (2017). What Are Omics Sciences?. In: Ametaj, B. (eds) Periparturient Diseases of Dairy Cows. Springer, Cham. https://doi.org/10.1007/978-3-319-43033-1_1
- Dai X, Shen L. Advances and Trends in Omics Technology Development. Front Med (Lausanne). 2022 Jul 1;9:911861. doi: 10.3389/fmed.2022.911861. PMID: 35860739; PMCID: PMC9289742.
- Rogers, Kara. “omics”. Encyclopedia Britannica, 20 Sep. 2024, https://www.britannica.com/science/omics. Accessed 22 September 2024.